Luminescence Resource Center
The glowing tail of a firefly or the bright blue waves of the ocean are some of the most familiar examples of luminescence in the natural world. Researchers recognized this phenomenon as a powerful platform to develop biological assays in the early years of biochemistry and molecular biology. In 1991, we released our first luciferase assay products, the first of many other assays that we have since built through innovative use of bioluminescent systems.
Learn more about how luminescence works, what makes it different from fluorescence and how you can use luminescence to fuel your own research.
Interested in luciferase reporter assays but not sure which one to choose? This guide will help you find the best one for your needs.
What is Bioluminescence?
Bioluminescence is a type of luminescence found in biological organisms where light is emitted due to a naturally catalyzed chemical reaction. These light-yielding reactions show up in organisms as diverse as fireflies, jellyfish, bacteria and more.
There are other types of luminescence as well, such as radioluminescence, which relies on radioactivity (e.g., scintillants) to produce light; and electroluminescence, which relies on electricity.
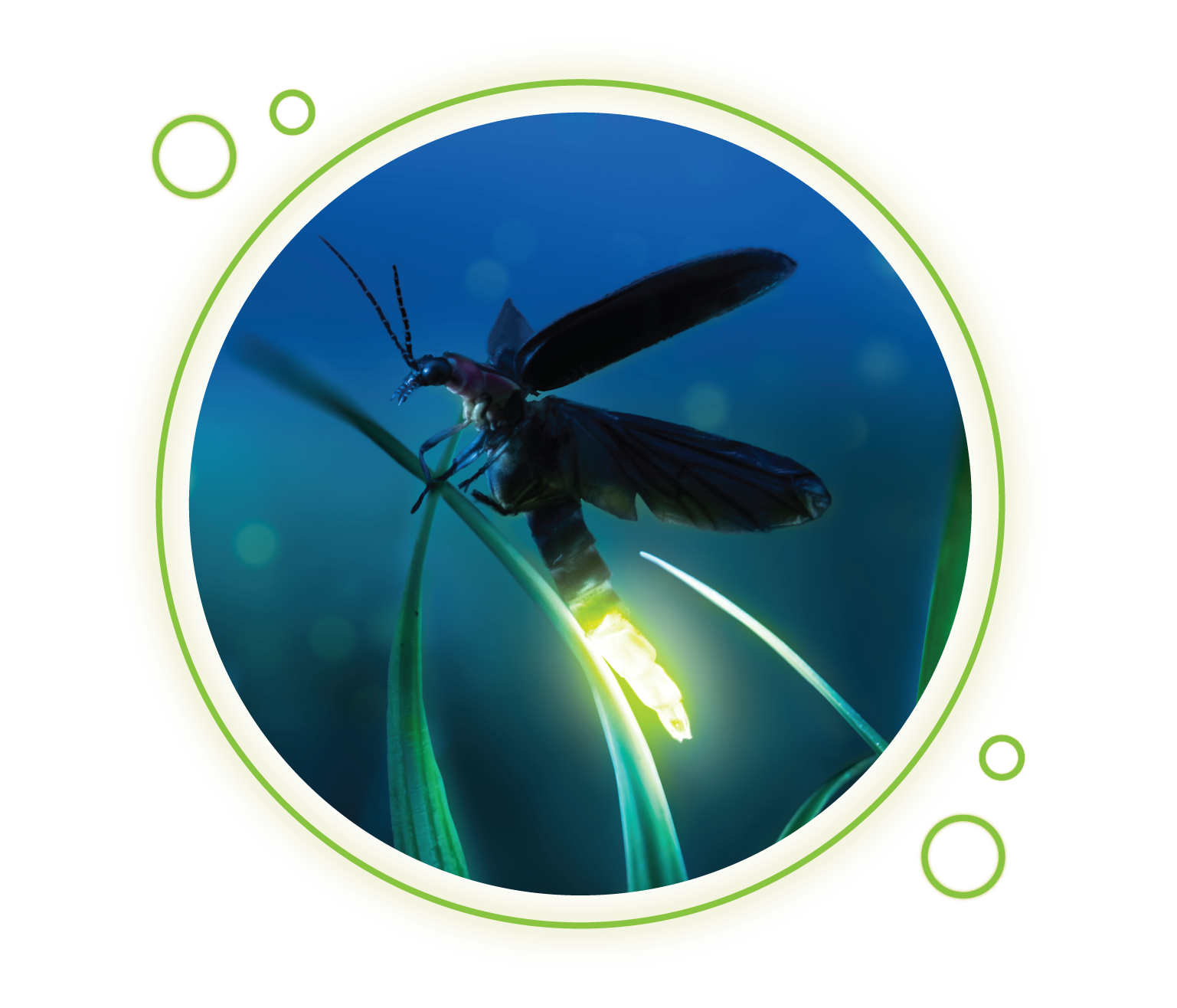
How Does Bioluminescence Work?
In a bioluminescent reaction, light is generated from chemical energy. A good example is the reaction catalyzed by the enzyme luciferase, which is the same reaction that makes fireflies glow. This reaction involves luciferin, oxygen (O2) and adenosine triphosphate (ATP) as the reagents. Luciferase and Mg2+ are catalysts for the reaction. Luciferase catalyzes the oxidation of luciferin to oxyluciferin. During the reaction, luciferin undergoes excitation to a more energetic chemical state. Then, the compound undergoes vibrational relaxation to a lower energy state. A final relaxation to ground state oxyluciferin releases energy in the form of light, a process called emission.


This luciferase-luciferin reaction is a canonical example of a native bioluminescent system. However, the native luciferase gene is not optimal for use in genetic reporter assays or other biological assays. In the 1990s, our scientists used genetic engineering to steadily optimize the native firefly luciferase gene. The final design offered a system where luciferase expresses uniformly and optimally in mammalian host cells, that minimizes off-target responses and that responds rapidly to transcriptional dynamics.
Other substrate and enzyme pairings can also generate bioluminescence. The color, intensity and duration of the emitted light will vary depending on the thermodynamics and kinetics of the specific reaction. For example, our scientists used directed evolution to transform a deep-sea shrimp’s bioluminescence system into our NanoLuc® luciferase system. Compared to native firefly luciferase, the reaction of NanoLuc® with its furimazine substrate is 100 times brighter.
The luminescent signal that results from a luminescent reaction can be coupled to any of the other reagents in the reaction. As an example, in a genetic reporter assay, the gene coding for luciferase is put under the control of the regulatory sequences from a gene of interest. When a reagent containing luciferin and ATP are added to the cell, the amount of light produced will be proportional to the amount of luciferase expressed. Higher gene expression results in greater luciferase expression and luminescent signal and vice versa. Other assays can measure the quantity or changes in ATP concentration or enzymatic release of luciferin or other luminescent substrates.
What’s the Difference Between Bioluminescence and Fluorescence?
Bioluminescence and fluorescence are both natural phenomena and are both regularly used in the lab as a tool for characterizing biological systems. The main difference between bioluminescence and fluorescence is the excitation source. Whereas chemical energy drives bioluminescence, photons drive fluorescence.
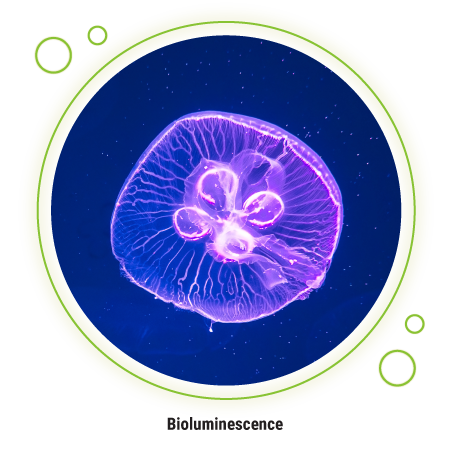
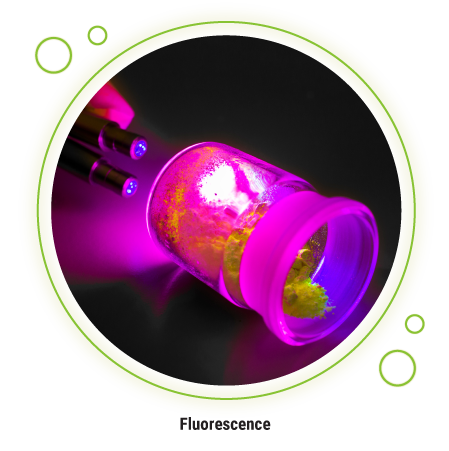
Fluorescent emissions can be very bright compared to luminescent emissions because the photons that drive excitation to the excited states can be introduced to a system at high rates. However, in biological assays, this influx of photons can also lead to higher backgrounds due to two factors: the need for the light detector to discriminate between excitation and emission photons and interference from other fluorescent groups in the sample.
For some techniques, like cellular microscopy and flow cytometry, instrument optics can restrict light so high backgrounds are not a problem. In these cases, the brightness of the system is the most important factor, and fluorescence is most commonly used.
For other cases where optical detection instruments are simpler and where there are more samples to analyze, lower background is needed. In those cases, luminescence can be a more valuable tool for biological assays.
What are the Benefits of Luminescent Assays?
Sensitivity
A primary advantage of bioluminescent assays is that they are generally very sensitive. Few eukaryotic cells produce their own light, so emission from a bioluminescent reaction, or signal strength, is much higher than any background signal. Bioluminescent assays, therefore, have very high signal-to-background ratios—bioluminescence can be routinely measured to levels as low as 10–20 moles. For a typical biological sample, that is equivalent to detecting only a few molecules per cell. This level of sensitivity is often compatible with endogenous expression levels, allowing for bioluminescent tools to accurately reflect native cellular biology.
Dynamic Range
The bright signal of bioluminescence assays allows for measuring a large dynamic range of bioluminescent emissions. Most bioluminescent assays give linear responses over six to eight orders of magnitude of substrate concentration. Fortunately, luminometers, the instruments of choice for quantifying bioluminescence, are capable of operating over these very large ranges.
High-Throughput Assays
The large linear dynamic range and corresponding sensitivity of bioluminescence allows for assays to be miniaturized and performed in medium to high-throughput plate formats, even 1536-well plates. Native bioluminescent reactions have been optimized by our scientists to enable quantitative light-based measurements that use “add-mix-measure” methods that simplify high-throughput assay formats.
Flexible Assay Design
The native firefly bioluminescent reaction gives a brief flash of light with a half-life of about 2 minutes. Our scientists have developed systems that yield longer-lived bioluminescent signals. In our flash-type assays, the signal half-life may only be about 10 minutes, but the signal is relatively bright. Our glow-type assays, in contrast, are less intense, but have signal half-lives often lasting several hours. These reagents can also be added directly to cells in culture, allowing for “add-mix-measure” assays that eliminate sample preprocessing and that are compatible with low- to high-throughput assay workflows.
Signal can be Coupled to a Range of Processes
Another benefit offered by bioluminescence assays is that light intensity generated by bioluminescent reactions is dependent on the concentrations of the component reactants. By coupling biological processes to these components, bioluminescent signals can be associated with a variety of molecular processes, including cell death, gene expression, protein-protein interactions and kinase activity.

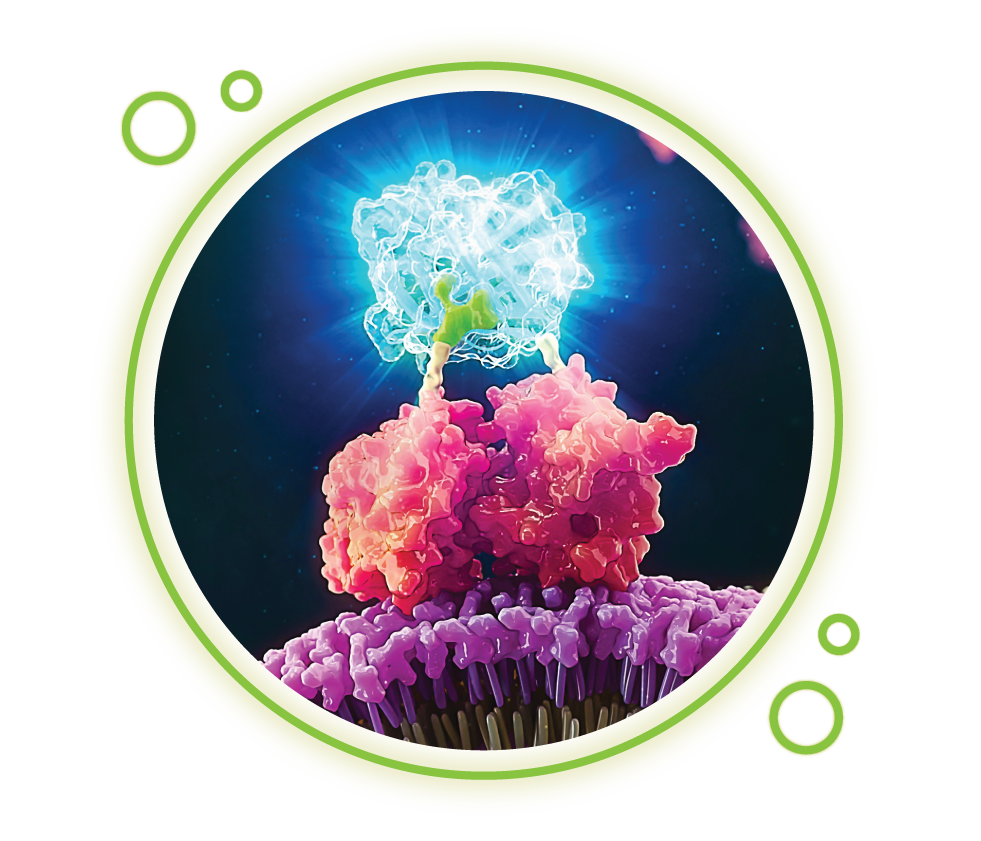
Wondering how bioluminescent assays could help answer questions in your research?
Talk with one of our scientists to learn what your options are. We have more than 30 years’ experience with bioluminescence and bioluminescent assays.
How Can You Use Luminescent Assays in Your Research?
At Promega, we’ve developed a variety of luminescent assays that can answer your specific research questions. Explore the resources below to find the assays you need.
Want to see the power of bioluminescence in action? Watch these videos to see how scientists transformed their research using bioluminescent assays.
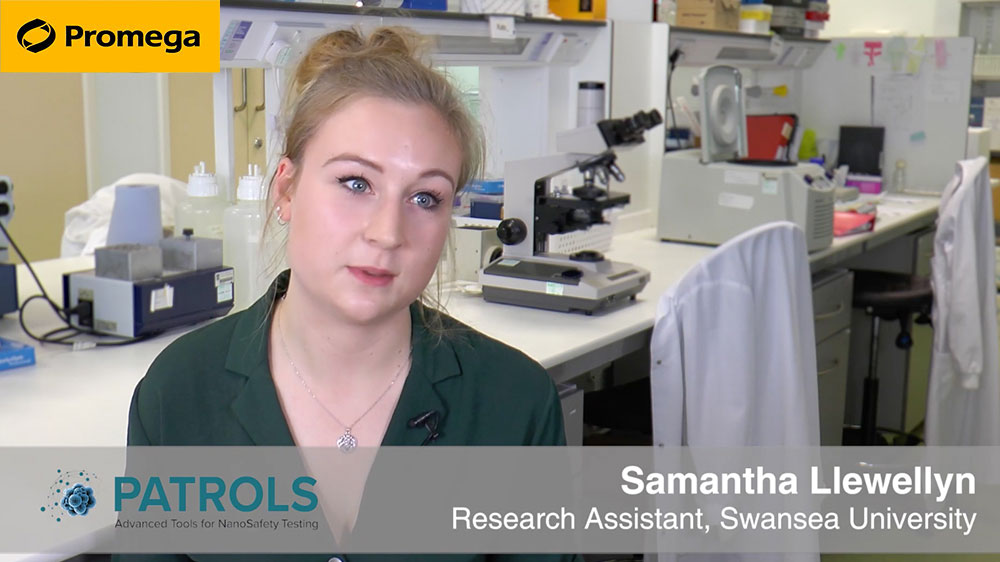
Developing Physiologically Relevant 3D Liver Models for HT Screening
Dr. Samantha Llewellyn at Swansea University uses the CellTiter-Glo® 3D assay to study her 3D cellular liver models. Learn more about bioluminescent assays optimized for use with 3D cell culture models with our Introduction to 3D Cell Culture guide.
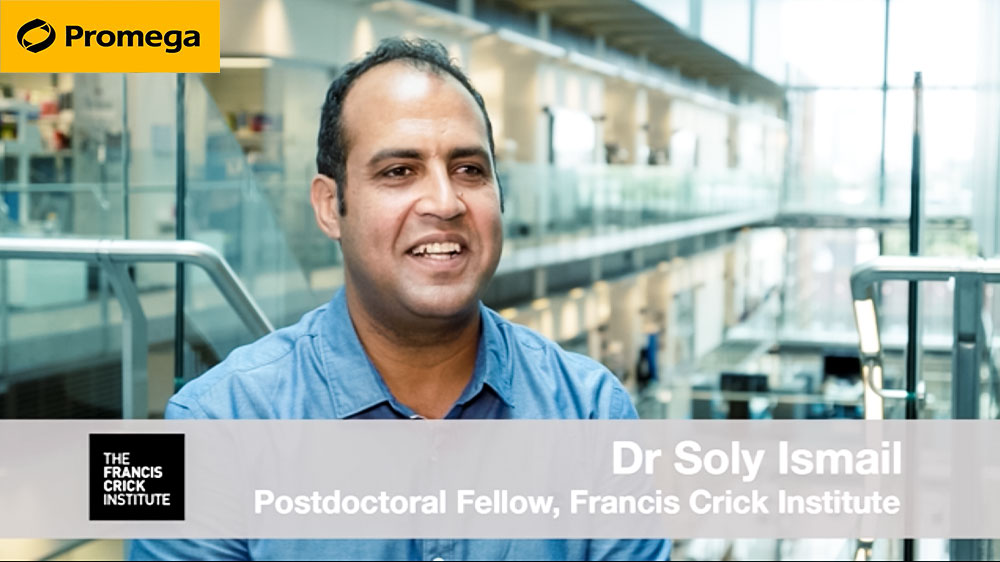
From Live Cells to Lysates: Adapting NanoBiT to a Biochemical Assay Format
Dr. Mohammad Ismail at the Francis Crick Institute uses a NanoBiT® Protein:Protein Interaction assay in a novel way to detect small molecule inhibitors for cancer drug discovery targets. Learn more about how our NanoBiT® technology was adapted to a biochemicalassay.
How Do You Measure Luminescence?
A luminometer is the most used instrument for measuring luminescence. They’re often simpler than instruments used to measure absorbance or fluorescence since they do not need a light source or filter, only a light detector. Luminometers usually don’t need to have a specific detection wavelength selected for an assay—they'll just collect light across the entire visible spectrum. This contributes to the strong signal-to-background you can achieve with luminescent assays.
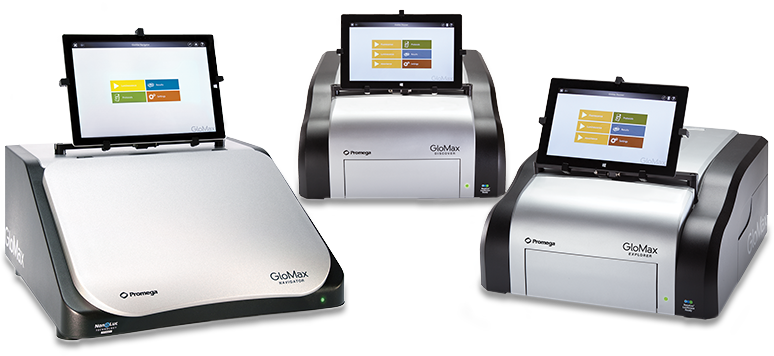
Luminometers can be in a plate reader or single tube reader format, and some instruments, like the GloMax® Discover and GloMax Explorer®, can measure luminescence, fluorescence and absorbance. Plate reader luminometers will have a light collector that measures the luminescent signal from individual microplate wells and will incorporate controls to prevent crosstalk, which occurs when light from one well bleeds over into adjacent wells.
There are two additional pieces of equipment you’ll need to carry out a luminescent assay.
If you’re using a microplate reader to analyze your assay, then you’ll need opaque white microplates, which reflect light and will maximize your assay’s signal. The bottoms of the wells can also be opaque or clear.
If you’re using a luminescent assay that produces a “flash-type” signal instead of a “glow-type” signal, then you’ll need one more piece of equipment—an injector. An injector will add substrate required to initiate a luminescent reaction and will coordinate the injection with signal collection from the luminometer.